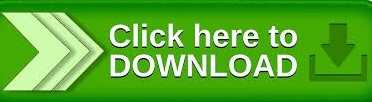

However despite many successes the ability of DE methods to identify variants with improvements in activity, sufficient for practical applications, is limited by the size of the libraries used versus the size of the sequence space they must search. This has led to the increased use of directed evolution (DE) methods to modify catalysis.
AUTOMUTE PROTEIN TRIAL
Currently the rational design of enhanced catalytic properties is largely based on qualitative judgements of protein structure and side chain chemistry, as well as heuristic rules based on trial and error. Predicting the effects of point mutations on catalysis is even more demanding, and there are currently no catalytic predictor algorithms equivalent to the PSPAs. Furthermore, comparative studies of the performance of several of these algorithms on large datasets of experimental ΔΔGfold values have not been able to clearly indicate why the algorithms fail in certain circumstances, nor suggest approaches to improve their accuracy. Despite achieving reasonable accuracy when comparing predicted and experimental stability changes averaged for a large number of mutant proteins, their accuracy is significantly lower when predicting individual point mutations, especially those involving structural features not well accounted for in the training datasets. Almost all the algorithms try to improve their accuracy by adjusting their individual energy terms with weights trained on experimental datasets of stability changes upon mutation (ΔΔGfold values).
AUTOMUTE PROTEIN FREE
These algorithms typically use either atom based energy functions or statistically based energy functions to predict the difference in free folding energy between the mutant and WT proteins. The effects of point mutations on conformational stability of a protein are often predicted using protein stability predictor algorithms (PSPA). Two properties that frequently require modification in practical applications of enzymes are increased thermodynamic stability and enhanced catalytic activity towards a desired substrate. The ability to engineer enzymes is of great importance both as a critical test of our understanding of the biochemistry involved and for practical applications in biotechnology and in particular biocatalysis. A feature vector for the I3A mutant only includes EC scores and supplemental information about the mutated position and the six closest neighbors determined by the lengths of simplex edges in (D). Virtually all the nonzero EC scores are negative, reflecting the impact of such a hydrophobic core residue replacement. Positions with nonzero EC scores correspond precisely to those whose Ca coordinates are vertices for the simplices in (D). (E) EC scores associated with the I3A mutant. All other residue positions represented at the vertices of these simplices by their respective Ca coordinates define the tessellation-based nearest neighbors of I3.
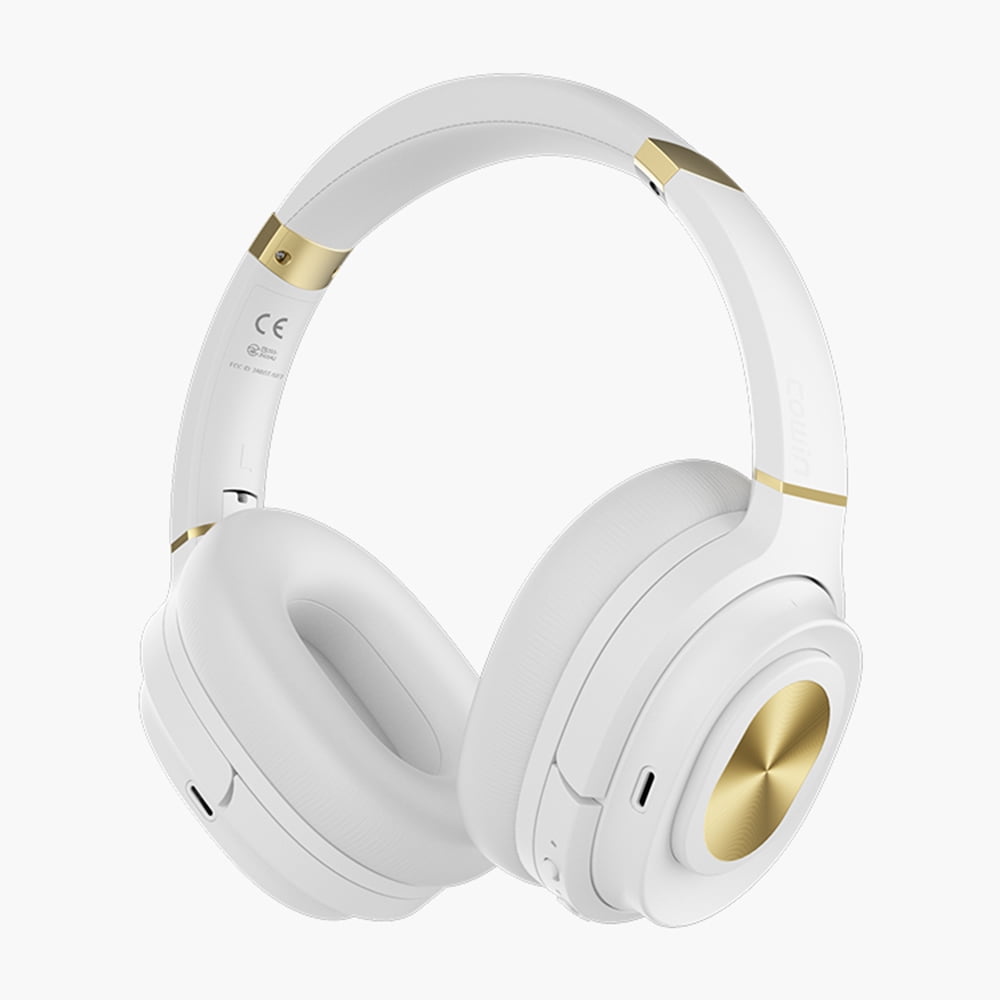
(D) Subset of tetrahedral simplices from the tessellation that share the Ca coordinate of residue I3 (enlarged relative to the others) as a vertex. (C) Delaunay tessellation of T4 lysozyme subject to a 12 A ˚ cutoff on allowable edge lengths.

(A) Ribbon diagram (Pettersen et al., 2004) and (B) Ca trace for bacteriophage T4 lysozyme based on the PDB coordinate file 3lzm.
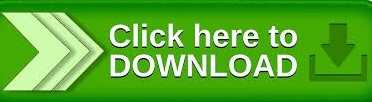